Hydrogen from electricity
Hydrogen is the lightest element in the periodic system and the most abundant chemical substance in the universe. It is usually foun in complex molecules such as fossil fuels like oil and gas or water.[i] Today, hydrogen in the EU is mainly used in oil and bio-refining (47%), ammonia production (39%), methanol production (8%) and industrial processing (4%)[ii].
​
Hydrogen can be produced in a process known as electrolysis by splitting water (H2O) into hydrogen (H2) and oxygen (O2) with electricity. There are various types of electrolysis, but the most commercial types of electrolysis currently on the market are alkaline and PEM (polymer electrolyte membrane) technologies[iii]. However, if the electricity used for the electrolysis is not low-carbon, then the hydrogen produced is not low-carbon either[iv].
​
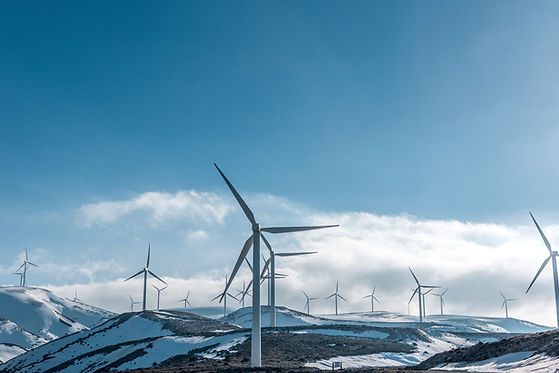
How it's made

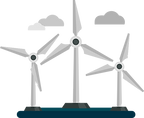
Climate impact
Low-carbon electricity is the most important condition for making low-carbon hydrogen.
When it burns, hydrogen produces energy without emitting greenhouse gases. However, the production of the hydrogen itself must be low-carbon for it to deliver emission reductions during its use. Only hydrogen produced with renewable electricity can be considered low-carbon. Once low-carbon hydrogen is produced, it can provide carbon-free heat, energy or act as an ingredient for other products and thus significantly reduce emissions from polluting sectors [v]. In industrial sectors, hydrogen can provide heat and energy, it can be used as a reducing agent for the steel sector or as a feedstock for chemicals [vi],[vii]. It can replace the high-carbon hydrogen currently used in for ammonia production (Haber-Bosch process), production of metals such as steel or copper and for the production of chemicals such as hydrogen peroxide.
​
The key ingredient for the production of low-carbon hydrogen production is an abundant supply of low-carbon and low-cost electricity. Hydrogen is only renewable when it is produced at the same time and place where the corresponding renewable electricity is generated. A temporal and geographical link between the renewables and the electrolysers producing hydrogen is imperative [viii]. Research suggests that electricity used for its production must be low carbon (less than 100 gCO2/kWh) for the hydrogen to be lower in emissions in comparison to conventional, fossil hydrogen that is derived from natural gas [1].
​
In order to include all emissions from the production of hydrogen with electricity, the full impact of the electricity source needs to be taken into account. For instance, materials and construction emissions from renewable electricity or emissions from fossil fuel use for electricity production in need to be counted [ix]. To prevent other environmental impacts, some low-carbon electricity sources shouldn’t be supported if the toll on the environment is too high (e.g. nuclear).
[1] Read more about our approach to quantifying the climate footprint of hydrogen in our positions on the EU Hydrogen Strategy and Sustainability Standards for Hydrogen from Electricity.
Hydrogen use
Due to the outlined limiting factors of availability and cost, hydrogen use should be focused. Since hydrogen will be made from electricity, it makes sense to use electricity directly when you can. For instance, it will achieve deeper reductions by replacing fossil hydrogen in the production of ammonia (i.e. fertiliser) rather than supplying fuel cells in the transport sector where a direct use of electricity for powering electric vehicles is much more efficient.
The scale of hydrogen needed, particularly in industry uses, can be large. To produce one tonne of raw steel with green hydrogen would require approximately 3.5 to 5 MWh of electricity[x],[xi],[xii],[xiii]. This means that the renewable electricity demand of a mid-sized steel mill producing 5 million tonnes of steel per year equals about 17.5 TWh. This is more than the entire annual electricity consumption of Berlin (13.5TWh).
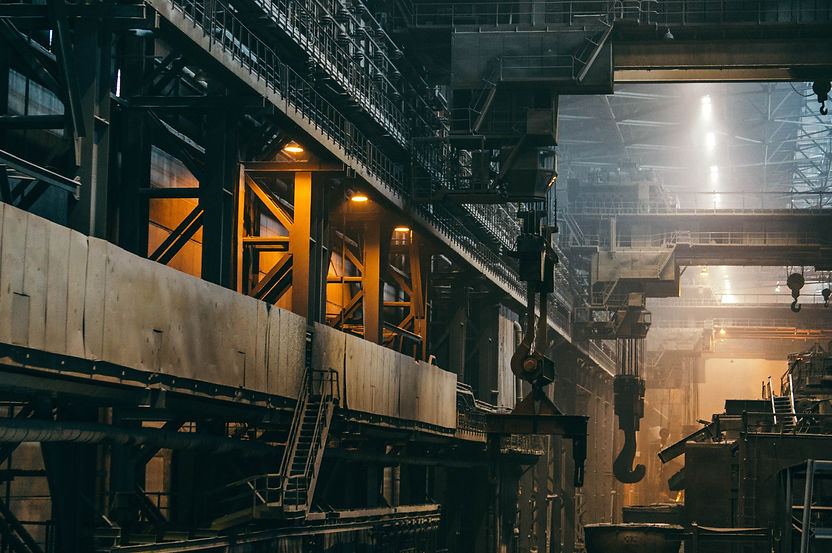
Infrastructure
A cross-border approach can significantly save costs and contribute to scaling up and rolling out a hydrogen infrastructure and the needed renewable energy associated. Additionally, hydrogen grids should be developed in a way does not obstruct electricity grid developments. By focusing on the direct electrification where possible and the use of hydrogen necessary, emission reductions can be maximised.[xiv]
​
Hydrogen can be stored either underground, in tanks or other suitable storage facilities. For distances shorter than 1500 km it is most cost effective to transport H2 by pipeline. For greater distances, transport via waterways becomes more affordable.[xv]
​
Climate score
Climate change mitigation

9/10

6/10
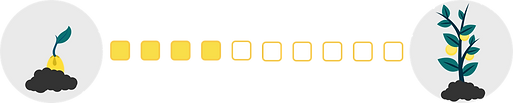
4/10
When very low-carbon electricity is used for the production and the hydrogen is used efficiently, electrolytic hydrogen can be almost carbon neutral, which is why it has a high climate change mitigation potential given certain conditions. It can also be produced at a large scale when renewable electricity becomes abundant.
Efficient use of resources
The conversion of electricity into hydrogen is resource intensive. If produced on an industrial scale, the production of clean hydrogen requires significant electricity and water resources.
Deployment readiness
While the efficiency of electrolysers has improved over the past few decades, deployment readiness in terms of the availability of low-cost and abundant renewable electricity sources is still low, contributing to the low score in this category.
Know of relevant research or projects to add to our library?
References
​
[i] Royal Society of Chemistry. 2020. Periodic table: Hydrogen. Available at: https://www.rsc.org/periodic-table/element/1/hydrogen
[ii] Fuel Cells and Hydrogen 2 Joint Undertaking. 2019. Hydrogen Roadmap Europe. Available at: https://www.fch.europa.eu/sites/default/files/Hydrogen%20Roadmap%20Europe_Report.pdf
[iii] J. Davies et al. 2020. Current status of Chemical Energy Storage Technologies, EUR 30159 EN, Publications Office of the European Union, Luxembourg, 2020, ISBN 978-92-76-17830-9, doi:10.2760/280873, JRC118776. Available at: https://publications.jrc.ec.europa.eu/repository/bitstream/JRC118776/current_status_of_chemical_energy_storage_technologies.pdf
[iv] Valente et al. 2020. Prospective carbon footprint comparison of hydrogen options. Science of The Total Environment (728). 1 August 2020, 138212. Available at: https://www.sciencedirect.com/science/article/abs/pii/S0048969720317253
[v] IRENA. 2019. Global energy transformation: A roadmap to 2050. Available at: https://irena.org/-/media/Files/IRENA/Agency/Publication/2019/Apr/IRENA_Global_Energy_Transformation_2019.pdf
[vi] TNO. 2020. Hydrogen as Raw Material for Industry and Fuel Transport. Available at: https://www.tno.nl/en/focus-areas/energy-transition/roadmaps/towards-co2-neutral-fuels-and-feedstock/hydrogen-for-a-sustainable-energy-supply/hydrogen-fuel-and-feedstock/
[vii] IES. 2018. Annex: Overview of breakthrough low-CO2 iron and steel production technologies. Available at: https://www.ies.be/files/ANNEX_Technology_Database_13072018.pdf
[viii] Directive (EU) 2018/2001 of the European Parliament and of the Council of 11 December 2018 on the promotion of the use of energy from renewable sources. 2018. OJ L 328 (90).
[ix] National Renewable Energy Laboratory. 2013. Life Cycle Greenhouse Gas Emissions from Electricity Generation. Available at: https://www.nrel.gov/docs/fy13osti/57187.pdf
[x] Bhaskar et al. 2020. Decarbonization of the Iron and Steel Industry with Direct Reduction of Iron Ore with Green Hydrogen. Energies 13(3). DOI: 10.3390/en13030758. Available at: https://www.researchgate.net/publication/339134196_Decarbonization_of_the_Iron_and_Steel_Industry_with_Direct_Reduction_of_Iron_Ore_with_Green_Hydrogen
[xi] Dolci, F., Green hydrogen opportunities in selected industrial processes - Workshop summary report (26th of June 2018, Centre Albert Borschette, Brussels, Belgium). 2018. EUR 29637 EN, Publications Office of the European Union, Luxembourg, 2018, ISBN 978-92-79-99135-6, doi:10.2760/634063, JRC114766. Available at : https://publications.jrc.ec.europa.eu/repository/bitstream/JRC114766/kjna29637enn.pdf
[xii] Vogl et al. 2018. Assessment of hydrogen direct reduction for fossil-free steelmaking. Journal of Cleaner Production 203: 736-745. DOI: Available at: https://www.sciencedirect.com/science/article/pii/S0959652618326301
[xiii] Krüger et al. 2020. Integration of water electrolysis for fossil-free steel production. International Journal of Hydrogen Energy. Available at : https://www.sciencedirect.com/science/article/pii/S0360319920331207
[xiv] Gasunie & Tennet. 2019. Infrastructure Outlook 2050, a joint study on integrated energy infrastructure in The Netherlands and Germany. Available at: https://www.tennet.eu/fileadmin/user_upload/Company/News/Dutch/2019/Infrastructure_Outlook_2050_appendices_190214.pdf
[xv] IEA. 2019. The Future of Hydrogen. Available at: https://www.iea.org/reports/the-future-of-hydrogen
[xvi] The Royal Society. 2018. Options for producing low-carbon hydrogen at scale. Available at: https://royalsociety.org/~/media/policy/projects/hydrogen-production/energy-briefing-green-hydrogen.pdf
​